
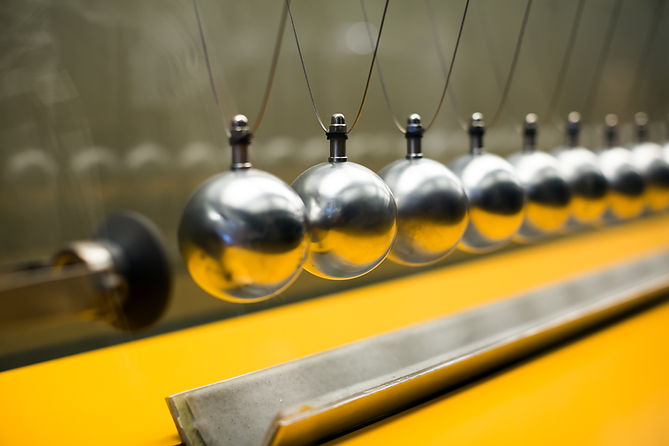
Research Areas
The lab explores the frontiers of engineering and natural systems, where critical transitions play a key role and the system can shift abruptly from one state to another. Such critical transitions can have undesirable or even catastrophic consequences including failure of space missions, power blackouts, extreme weather, extinction of species in ecosystems and sudden crash of financial markets.
​
We study transitions in three flow systems using the framework of complex systems theory:
-
Oscillatory instabilities in thermofluid machinery with turbulent flow;
-
Extreme events in climate systems and their cascading effect;
-
Onset of precipitation in clouds.
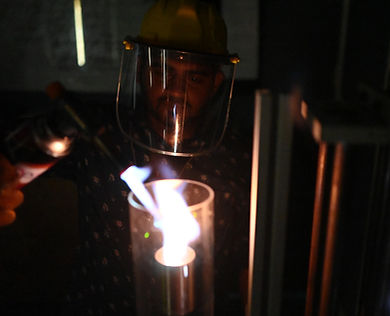
Thermoacoustics
Feedback between turbulent flow and other subsystems is often the cause for oscillatory instabilities. The nonlinear feedback between the reactive flow field and the acoustic field in an engine gives rise to thermoacoustic instability, a state of ruinously large-amplitude periodic oscillations. This phenomenon can cause structural damages due to the increased thermal and vibrational loads, forcing shutdown of gas turbine engines, or failure of rockets.
Precipitation and turbulence in clouds
Clouds have a significant effect on weather. Clouds are water vapor in the atmosphere that has condensed to a dispersion of droplets and ice particles interacting with a complex turbulent flow. They are inhomogeneous, intermittent, highly non-stationary and comprise an enormous range of spatial and temporal scales with strong couplings across these scales. Clouds are complex systems which play a key role in emergent phenomena such as rain, tornadoes, cyclones and even the climate system. Complex systems theory presents us with an opportunity to study how small-scale processes such as condensation, droplet coalition etc. in clouds couple to the flow at large scales and how this coupling affects the weather and climate.
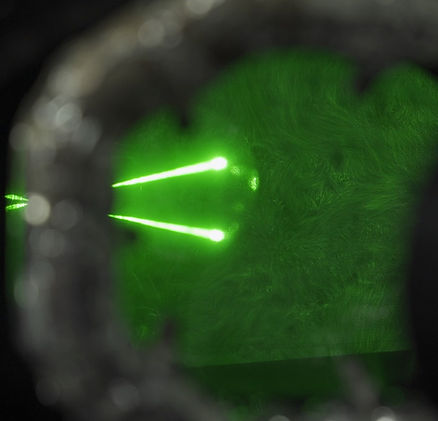

Climate dynamics
The Earth’s climate is a complex system, i.e., it consists of multiple sub-systems, such as fluid flows, heat transfer, and thermodynamics, among many others that interact with each other across multiple time and length scales. These interactions are complex in nature, which enhances the variability of the climate system making it less predictable. Furthermore, the long range interactions, also known as teleconnections, are responsible for extreme weather events such as excessive rainfall, droughts, formation and merging of cyclones. In our group, we study phenomena ranging from micro-scale where flow turbulence plays a major role (hyperlink to cloud team), synoptics scale (tropical cyclones) to planetary scale (intertropical convergence zone) using the framework of complex networks. We develop novel methods to quantify interactions that are based on physical phenomena and novel network measures to bring out patterns of teleconnections in the climate. Through such analysis we develop relevant climate-based region classification, develop precursors for cyclone merging, cyclone tracking and prediction of extreme precipitation.